The dawn of quantum computing heralds a transformative era across myriad industries, promising to tackle computational behemoths at speeds unfathomable to classical computers. This technological marvel leverages the principles of quantum mechanics, opening a pandora’s box of capabilities that could catapult industrial efficiency into a new stratosphere.
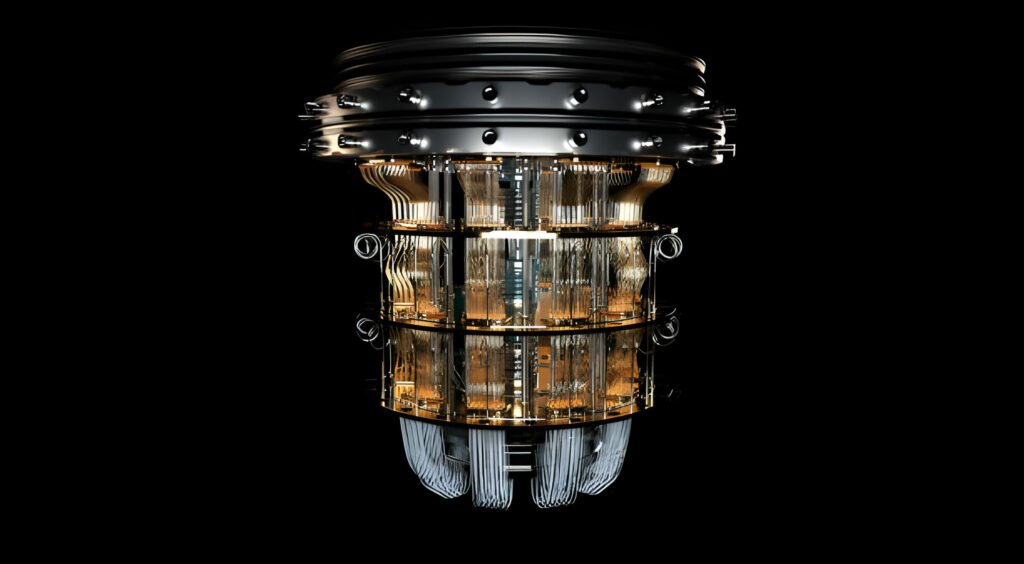
Image Credit: iStock.com
Table of Contents
Quantum Computing - An Overview
At its core, quantum computing departs from binary code, utilizing quantum bits or ‘qubits’. Unlike binary bits that represent either a 0 or 1, qubits can exist in multiple states simultaneously through superposition. Additionally, entanglement, a phenomenon where qubits become intrinsically linked, ensures that the state of one instantly influences the other, irrespective of distance. This quantum ballet facilitates formidable computational power, enabling quantum computers to process complex datasets at groundbreaking speeds.
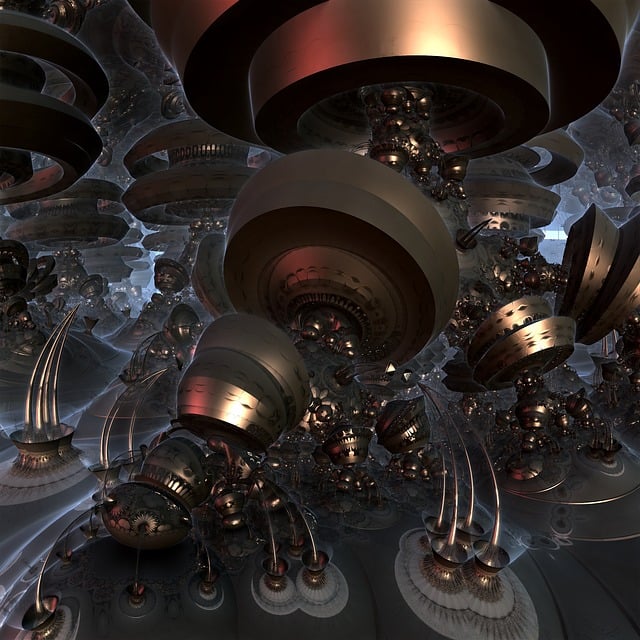
Image Credit: Pixabay.com
Unveiling the Era of Quantum Innovation
Reimagining Data’s Future:
Poised at the brink of a data revolution, quantum computing bursts onto the scene, primed to redefine data handling and synthesis. Immersed in the nuances of quantum theory, its roots run deep into the fertile grounds of quantum physics, empowering it to decipher riddles far beyond the reach of traditional computing.
This next-generation powerhouse stands ready to churn through labyrinthine calculations with a swiftness that makes “real-time” seem sluggish. Picture an era where businesses manipulate oceans of data as effortlessly as a sculptor shapes clay. That’s the promise of quantum computing—data processing transformed into an art form as it flows, melds, and emerges anew with astonishing velocity and precision.
In the near horizon, quantum computing looms as the pivotal force in reshaping how we merge, mold, and mine data. It’s an alchemist in the digital age, poised to transmute raw data into golden insights.
Quantum computing rests on three core principles: Superposition, Qubits, and Entanglement
Superposition:
Superposition describes a quantum system’s capacity to be in multiple states simultaneously. Picture an electron, which can exhibit a ‘spin’ — imagine it like an arrow that can point either up (right) or down (left). Classical physics would demand the electron choose a direction, but in quantum mechanics, it can be in a superposition, where it’s essentially spinning both ways at once. It’s only when we measure it that the electron ‘decides’ on a clear state, a phenomenon known as ‘collapse.’Â
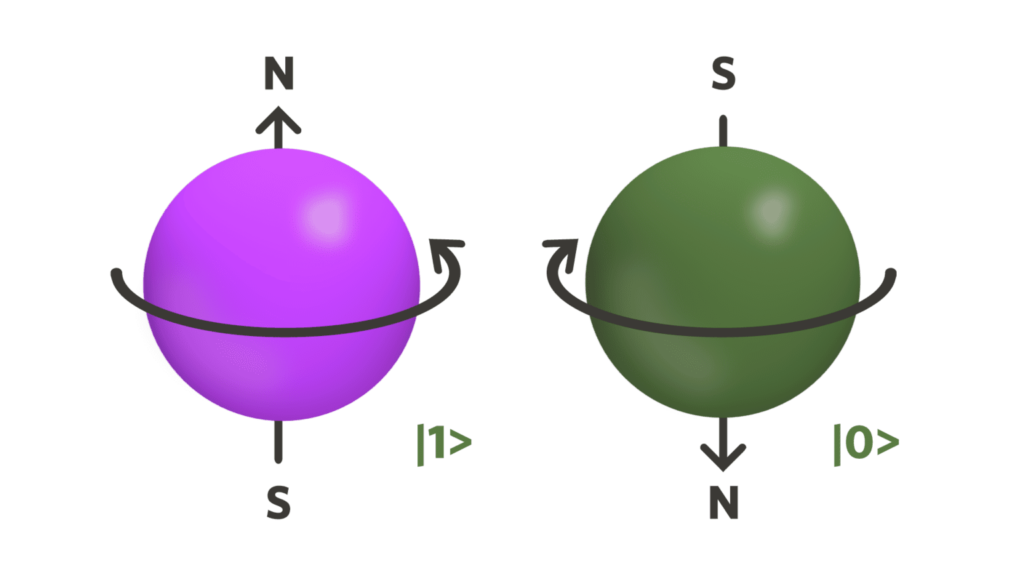
In computing terms, if a traditional bit holds a steadfast 0 or 1, its quantum counterpart — the qubit — can hold a 0, a 1, or any quantum superposition of these states. This duality alludes to much richer information potential before a measurement pins it down.
Â
Â
Qubits:
Qubits are the quantum analogue of classical bits, but they harness superposition. In classical computers, bits are the fundamental unit of data, strictly being either 0 or 1. Qubits, however, can take a value of 0, 1, or both simultaneously due to superposition. They’re typically represented by the symbols |0> and |1>, which in quantum mechanics refer to the different states a qubit can have.Â
When many qubits are in superposition, they can represent a vast number of possible states at once, allowing quantum computers to process complex problems at speeds unreachable by classical machines.
Â
Entanglement:
Entanglement is a uniquely quantum phenomenon where two particles become linked, so the state of one (whether spin, position, momentum) instantaneously influences the state of the other, regardless of the distance between them. If two qubits are entangled, knowing the state of one immediately informs us about the state of the other. This link remains steadfastly in place whether the particles are next to each other on a chip or at opposite ends of the universe.Â
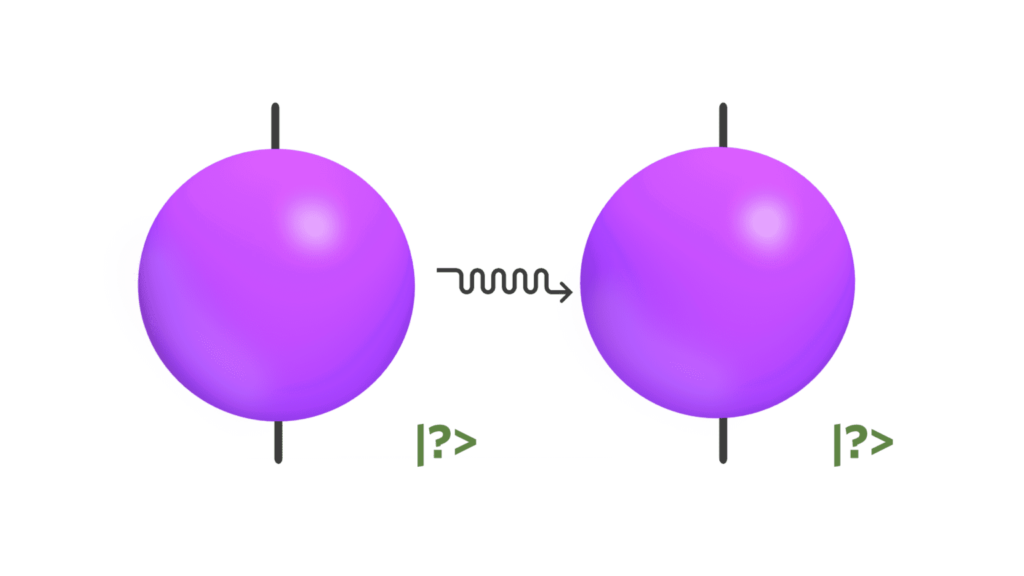
Entanglement enables quantum computers to tackle problems in novel ways. For instance, paired qubits in an entangled state can perform calculations in tandem, almost as if they are communicating instantaneously. It’s this property that helps facilitate quantum speedups — the astonishing pace at which quantum processors can perform certain calculations compared to classical processors.
Together, superposition and entanglement allow quantum computers to explore a multitude of computations simultaneously. This multi-layered exploration is why quantum computers hold such promise for complex tasks like simulating molecular interactions for drug discovery, cracking cryptographic codes, or sifting through vast databases at speeds beyond classical capabilities.
Â
While enthralling, these quantum principles also impose new challenges, such as error rates and decoherence, where qubits lose their quantum properties. The nascent technology of quantum computing continues to evolve, with these three concepts — superposition, qubits, and entanglement — being the bedrock on which it stands and the source of its potential to revolutionize computing.
Quantum Cloud Services: Pioneering Access to the Future of Computing
Access to cutting-edge quantum computing hardware is now available through some large cloud service providers, each offering distinct platforms and services designed to harness quantum computing’s potential. Let’s delve into the offerings and hardware specifics.
Â
Google has launched the Quantum AI lab where they make use of quantum processors like Bristlecone and more recently, Sycamore. Google’s Sycamore is a notable processor with 53 qubits that famously performed a computation in 200 seconds that would have taken the world’s most powerful supercomputer 10,000 years to complete, as claimed by Google.
Â
Microsoft’s contribution to this field is encapsulated in their Quantum Development Kit, which is more focused on the software side, providing developers tools to write quantum code. However, Microsoft collaborates with quantum hardware manufacturers and is deeply invested in developing topological qubits, which are considered to be much more stable than other types.
Â
Amazon’s AWS Braket service allows users to design quantum algorithms, test them on simulated quantum computers, and run them on different types of actual quantum hardware. The AWS Braket hosts quantum hardware from different vendors, thus users can select the preferred underlying quantum computation technology.
Â
IBM, a prominent player in the quantum space, offers the IBM Quantum Experience, which allows users to run experiments on IBM’s quantum processors. IBM is aggressively pursuing a roadmap to increase its qubit count, planning for processors with 128 qubits and beyond. This advancement is significant as it would dramatically increase the computational capabilities of their systems.
Quantum Hardware Infrastructure
In terms of the hardware infrastructure, vendors like D-Wave, IonQ, and Xanadu are pioneering. D-Wave is known for their quantum annealers, like the 2000Q which utilizes a different approach from the quantum gate model for solving optimization problems. IonQ, on the other hand, uses trapped ion technology for their quantum processors, which emphasizes precision and long coherence times. Xanadu’s approach is unique as they focus on photonic quantum computing, leveraging the properties of light for quantum computation.
Â
Â
The “qubit count,” which you mention, is one of the key metrics in evaluating quantum computers because it indicates the system’s potential complexity and processing power. Quantum systems with more qubits can theoretically solve more complex problems. However, it’s not just about the number of qubits. The quality of the qubits, their coherence times, gate fidelities, and error rates are critical metrics for practical use.
Â
Â
The quantum computing landscape is evolving rapidly, with advancements expected in both qubit counts and overall system performance. As higher qubit processors become available, the complexity of problems that quantum computers can tackle will scale, potentially revolutionizing fields like cryptography, materials science, and optimization. The transition to mainstream quantum computing brings with it challenges and the excitement of stepping into a new era of computational possibilities.
Unraveling the Complexities: The Roadblocks to Quantum Computing's Widespread Adoption
Breaking down the challenges that hinder the mainstream adoption of quantum computing uncovers a web of scientific and practical hurdles that researchers and engineers face.
Stability: The foundation of quantum computing—the qubit—is notoriously unstable due to quantum decoherence, which occurs when qubits lose their quantum state due to interaction with the environment. This is often exacerbated by heat and electromagnetic waves. Qubits need to operate at near absolute zero temperatures to remain stable, requiring sophisticated refrigeration technology. Reducing error rates and developing fault-tolerant quantum computers are currently major research areas.
Scalability: Adding more qubits increases the computational power, but it also amplifies the difficulty in maintaining their stable operation. Manufacturing challenges also arise when scaling up: creating identical, high-quality qubits becomes harder as their number grows. While dozens of qubits have been managed in lab settings, scaling up to thousands, which would be necessary for broad-spectrum applications, presents a significant technical hurdle.
Connectivity: The definition of quantum computers revolves around the concept of entangled particles. Establishing these connections, or entanglements, between increasingly larger numbers of qubits is complex. This intricacy grows exponentially, not linearly, with each additional qubit due to the nature of quantum states, posing a serious issue for expanding quantum networks.
Decoherence: Decoherence is related to stability but deserves its own mention as a significant obstacle. As qubits transition from superposition states (where they hold both 0 and 1 simultaneously) to a single state, useful information can be lost. This transition naturally happens over extremely brief time frames, and maintaining coherence long enough for complex calculations remains a demanding task.
Programming: Quantum algorithms demand an entirely different approach than classical computing. Software programs that run on quantum computers need to be designed to exploit quantum phenomena like superposition and entanglement. This requires programmers both to grasp quantum mechanics to a certain extent and to think in a non-intuitive way compared to classical software design. Current computer science educations and tools are not sufficient to handle the peculiarities of quantum programming.
Standardization: Just like with classical computing, widespread adoption of quantum computing requires standardized models for software and hardware interaction. Establishing such standards will likely be a gradual process, informed by both technical breakthroughs and industry consensus. There’s no clear leader in the field yet to set these standards, and methodologies remain diverse.
Access and Availability: Entry to quantum computing is costly. Sophisticated equipment, ultra-cold operating environments, and the expertise needed to program and operate quantum machines put them out of reach for most organizations. Access is currently limited to high-end research institutions or very large businesses that can afford to experiment with the technology.
The industry is addressing these formidable challenges. Advances in error correction codes, quantum materials, cryogenics, quantum software development, and innovative system architectures are being researched and developed. Overcoming these barriers will require a combination of scientific progress, engineering ingenuity, and substantial investment. The future of quantum computing is premised on navigating these obstacles; success here will unlock unprecedented computational capabilities.
Unlocking Future Capabilities: Diverse Advantages of Quantum Computing Across Industries
1. Pharmaceuticals and Healthcare: Quantum computing could expedite drug discovery by analyzing molecular structures and interactions with unprecedented precision. This means faster time-to-market for life-saving drugs and a revolution in personalized medicine.
2. Finance: Optimizing portfolios, risk assessment, and fraud detection could be revolutionized as quantum computing manages vast, complex financial systems and market data at lightning-fast speeds.
3. Energy: In the energy sector, quantum computing could significantly enhance battery design, energy distribution, and contribute to the discovery of new, sustainable materials.
4. Logistics: Tackling the traveling salesman problem on a grand scale, quantum computing could optimize routing for global logistics networks, saving time and resources.
5. Artificial Intelligence: Quantum computing can supercharge AI algorithms, leading to advances in machine learning and, consequently, more intelligent and efficient AI.
Challenges Ahead
Despite the bright prospects, practical quantum computing faces significant hurdles. Qubits are notoriously sensitive to external disturbances, and maintaining coherence for substantial periods remains a colossal challenge. As quantum technology matures, cybersecurity becomes a paramount concern, needing novel encryption techniques such as quantum cryptography.
Conclusion
Quantum computing is no mere theoretical wonder; it is a beacon of industrial revolution. As it strides from lab to real-world applications, it holds the promise of solving complex calculations at speeds that will redefine what is computation. The industries that harness this power stand on the brink of an unprecedented competitive edge. The quantum future isn’t just coming; it’s nearly upon us, and it’s poised to redefine the technological landscape of our time.
FAQs : Quantum Computing
Quantum computing harnesses quantum mechanics principles like superposition and entanglement to process information. Unlike classical bits, which are either 0 or 1, quantum bits (qubits) can be in multiple states simultaneously, enabling quantum computers to solve complex problems much faster.
While still in the development phase, in the future, quantum computing could lead to breakthroughs in material science, personalized medicine, and optimization problems, indirectly improving products and services for consumers.
Industries like pharmaceuticals, materials engineering, finance, cyber security, and even complex systems modeling like climate prediction are poised to be revolutionized by quantum computing capabilities.
Yes, though mainly at a research level or via cloud platforms offered by companies like IBM, Google, and others. These are not yet as powerful as the anticipated future quantum computers but are valuable for developmental research and testing.
Quantum computing presents both risks and opportunities: it could potentially break current encryption methods, but it also promises more secure communication through quantum cryptography.
There is no fixed timeline, as developments are rapid but also complex. Some industry experts suggest we could have more advanced and practical quantum computers within this decade, but estimates vary widely.
Businesses can start by educating themselves about the technology, partnering with quantum technology firms, investing in quantum-ready cybersecurity, and beginning to integrate quantum algorithms into their operations where feasible.
Quantum computing has the potential to process vast and complex datasets more efficiently, which could greatly enhance AI and machine learning capabilities, leading to more powerful predictive models and algorithms.
Quantum computing currently faces issues like error rates, qubit coherence times, and the need for extremely low temperatures, making the technology challenging to scale and maintain.
Quantum supremacy refers to the point at which a quantum computer can perform a calculation that no classical computer can achieve in a feasible time frame. It’s a milestone indicating significant progress in quantum computing performance.