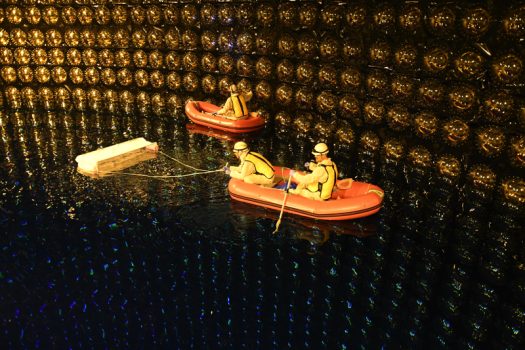
Kamioka Observatory, ICRR (Institute for Cosmic Ray Research), The University of Tokyo
Buried 1,000 meters under Mount Ikeno in Japan, the Super-Kamiokande is not just any underground facility. It looks like something out of a sci-fi movie—a giant tank of ultra-pure water surrounded by thousands of golden light detectors. But this isn’t for show. It’s one of the most advanced neutrino detectors on the planet, built to study ghost-like particles that pass through everything, including us, without leaving a trace.
The underground location isn’t just for secrecy. The thick rock shields the detector from cosmic radiation, ensuring that only neutrinos are observed. This is crucial because neutrinos are nearly impossible to detect. They don’t interact with matter like other particles do. But when they occasionally collide with water molecules in the tank, they create tiny flashes of light. Scientists use these flashes to study neutrinos, revealing clues about dying stars, black holes, and the universe itself.
Super-Kamiokande has been running since 1996 and continues to push the boundaries of particle physics.
Table of Contents
What Are Neutrinos and Why Do They Matter?
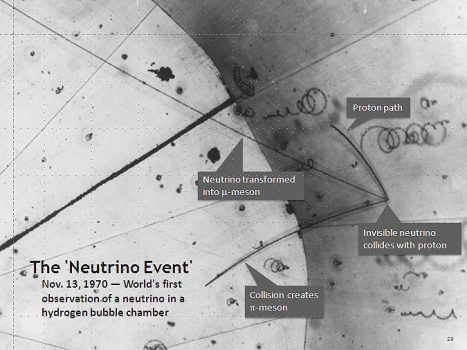
Argonne National Laboratory, Public domain, via Wikimedia Commons
Neutrinos are one of the most mysterious particles in the universe. They have no electric charge, barely any mass, and pass through solid objects like they aren’t even there. Every second, trillions of them travel through your body without interacting with a single atom. This makes them extremely difficult to detect, but also incredibly important to study.
Scientists believe neutrinos hold answers to some of the biggest mysteries in physics. They come from nuclear reactions inside stars, exploding supernovae, and even the Big Bang itself. By studying neutrinos, researchers can understand what’s happening inside stars, how the universe evolved, and why matter exists at all.
Super-Kamiokande plays a key role in neutrino research. The detector captures rare interactions between neutrinos and water molecules, allowing scientists to study their properties. One of the biggest discoveries made at Super-Kamiokande was that neutrinos can change from one type to another—proving they have mass. This breakthrough changed our understanding of physics and won the 2015 Nobel Prize in Physics.
How Super-Kamiokande Detects the Invisible
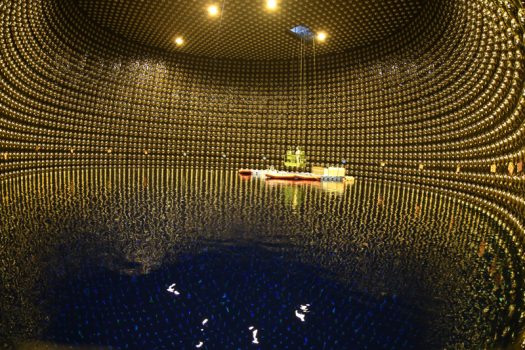
Kamioka Observatory, ICRR (Institute for Cosmic Ray Research), The University of Tokyo
Detecting neutrinos is like trying to catch a single grain of sand in a hurricane—it’s almost impossible. But Super-Kamiokande has found a way. The key is its massive underground water tank, which holds 50,000 tons of ultra-pure water. This water acts as a target for neutrinos passing through it.
When a neutrino interacts with a water molecule, it creates a faint blue flash of light called Cherenkov radiation. These flashes are detected by over 11,000 photomultiplier tubes (PMTs) lining the tank’s walls. These PMTs are highly sensitive light detectors that amplify the signal and help scientists analyze the neutrino’s direction, energy, and type.
The location deep inside Mount Ikeno is critical. It blocks out unwanted noise from cosmic rays, ensuring only neutrino interactions are recorded. Without this shielding, the data would be contaminated by background radiation from space. This setup has made Super-Kamiokande one of the most precise neutrino detectors in the world, allowing groundbreaking discoveries in astrophysics and particle physics.
The Discovery That Changed Physics Forever
One of Super-Kamiokande’s biggest breakthroughs was proving that neutrinos have mass. For decades, scientists believed neutrinos were massless, like photons. But something wasn’t adding up—experiments kept detecting fewer neutrinos from the Sun than expected. This became known as the “solar neutrino problem.”
In 1998, Super-Kamiokande solved this mystery. Scientists discovered that neutrinos don’t just travel in a straight line—they oscillate, or change from one type to another as they move. This was groundbreaking because, according to physics, only particles with mass can oscillate. The discovery rewrote fundamental theories and earned a share of the 2015 Nobel Prize in Physics.
This wasn’t just a small technical detail. It changed how scientists understand the universe. It hinted that the Standard Model of particle physics—one of the most successful theories ever—was incomplete. Neutrinos might hold clues to new physics beyond what we currently understand, opening the door to discoveries about dark matter, antimatter, and even the origins of the universe itself.
How Super-Kamiokande Detects Exploding Stars
One of the most exciting roles of Super-Kamiokande is detecting supernovae—massive star explosions that send shockwaves across the universe. When a star dies, it releases an enormous burst of neutrinos, which travel through space almost unaffected by anything in their path. Because neutrinos move faster than the visible explosion itself, they can act as an early warning system for astronomers.
In 1987, a previous version of Super-Kamiokande, called Kamiokande-II, detected neutrinos from a supernova in the Large Magellanic Cloud. This was the first time neutrinos from a supernova had been observed on Earth. It confirmed that most of a supernova’s energy is carried away by neutrinos and gave scientists new insights into how stars die.
Today, Super-Kamiokande is part of the Supernova Early Warning System (SNEWS). If it detects a sudden burst of neutrinos, it alerts astronomers worldwide, giving them time to point telescopes at the right place in the sky before the explosion becomes visible.
The Role of Super-Kamiokande in the Search for Dark Matter
Dark matter is one of the biggest unsolved mysteries in physics. Scientists believe it makes up about 27% of the universe, yet no one has ever seen it. It doesn’t emit light, interact with normal matter in any obvious way, or leave a clear trace. But researchers suspect neutrinos might hold the key to unlocking its secrets.
Super-Kamiokande is helping in this search by studying neutrinos that might be linked to dark matter. Some theories suggest that dark matter particles could occasionally decay into neutrinos. If this happens, the detector could pick up unusual neutrino signals that don’t match those from known sources like the Sun or cosmic rays.
By analyzing these signals, scientists hope to learn more about dark matter’s nature and behavior. Even though no direct evidence has been found yet, every new piece of data brings researchers closer to understanding what dark matter is and how it shapes the universe. Super-Kamiokande remains at the forefront of this search.
Upgrades and the Future of Super-Kamiokande
Super-Kamiokande has undergone several upgrades to improve its sensitivity and expand its research capabilities. One of the most significant upgrades was in 2018, when researchers added gadolinium sulfate to the water. This rare element enhances the detector’s ability to distinguish neutrinos from different sources, particularly those from supernovae.
Another major development is the planned Hyper-Kamiokande project, a next-generation neutrino detector that will be even larger and more advanced. It will hold nearly 200,000 tons of ultra-pure water—four times the size of Super-Kamiokande—and have more powerful photomultiplier tubes. This will allow scientists to detect neutrinos with unprecedented precision.
These upgrades mean Super-Kamiokande will continue making discoveries for years to come. It will help scientists refine their understanding of neutrino properties, improve predictions about supernovae, and possibly detect signs of new physics. As technology advances, this hidden lab under Mount Ikeno will remain one of the world’s most important tools for exploring the universe’s deepest mysteries.
Why Super-Kamiokande Is Buried Underground
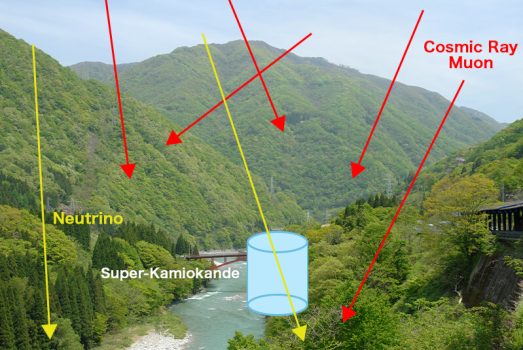
Kamioka Observatory, ICRR (Institute for Cosmic Ray Research), The University of Tokyo
Super-Kamiokande’s location 1,000 meters under Mount Ikeno isn’t just for secrecy—it’s essential for the experiment to work. The thick rock above acts as a natural shield, blocking out cosmic rays and other background radiation that could interfere with neutrino detection.
On the surface, cosmic rays constantly bombard the Earth, creating noise that would overwhelm delicate neutrino signals. By placing the detector deep underground, scientists can filter out most of this interference, allowing them to study neutrinos with much greater accuracy.
The underground setting also provides a stable environment. The temperature and pressure remain constant, which helps maintain the ultra-pure water inside the detector. Even the smallest contamination could affect the results, so every precaution is taken to keep the environment pristine.
Without this underground isolation, detecting neutrinos would be nearly impossible. The location gives Super-Kamiokande a level of precision that few other experiments can achieve, making it one of the most reliable tools for studying these elusive particles.
Super-Kamiokande’s Impact on Astrophysics and Cosmology
Super-Kamiokande isn’t just a particle physics experiment—it has changed how scientists understand the universe. By studying neutrinos, researchers have gained insights into the life cycles of stars, the nature of matter, and even the origins of the cosmos.
One of its biggest contributions is in supernova research. By detecting neutrinos from dying stars, Super-Kamiokande has provided crucial data on how massive stars collapse and form neutron stars or black holes. This has helped refine models of stellar evolution and energy distribution in the universe.
The detector has also contributed to the study of cosmic rays, high-energy particles that travel through space at nearly the speed of light. By analyzing neutrino interactions, scientists can trace the origins of these cosmic rays and understand their impact on galaxies, including our own Milky Way.
As new discoveries continue to emerge, Super-Kamiokande remains a vital tool in astrophysics, bridging the gap between particle physics and the study of the universe’s most extreme phenomena.
The Legacy of Super-Kamiokande and What Lies Ahead
Super-Kamiokande has already made history, but its journey is far from over. With continuous upgrades and future projects like Hyper-Kamiokande on the horizon, the search for answers about neutrinos, dark matter, and the universe’s origins will only intensify.
The detector’s discoveries have reshaped fundamental physics, proving that neutrinos have mass and challenging the Standard Model. It has helped scientists understand supernovae, cosmic rays, and even the possible existence of new physics beyond what we currently know.
As technology advances, Super-Kamiokande’s role will continue to expand. Whether it’s detecting the next supernova before it lights up the sky or uncovering hidden properties of neutrinos, its impact on science will be felt for decades to come.
This underground lab, hidden beneath a mountain in Japan, is one of humanity’s greatest scientific achievements. It serves as a reminder that even the most elusive mysteries can be unraveled with patience, innovation, and a deep curiosity about the universe.
FAQs
Super-Kamiokande is a neutrino detector used to study neutrinos, detect supernova explosions, and explore fundamental physics. It helps scientists understand the properties of neutrinos, search for dark matter, and investigate cosmic rays.
It uses a massive tank filled with 50,000 tons of ultra-pure water. When a neutrino interacts with a water molecule, it produces a faint flash of light called Cherenkov radiation. Thousands of photomultiplier tubes (PMTs) detect these flashes, allowing scientists to analyze neutrino properties.
It is buried 1,000 meters under Mount Ikeno to block cosmic radiation. The rock above acts as a natural shield, preventing interference from other particles and ensuring accurate neutrino detection.
Its most significant discovery was proving that neutrinos have mass by detecting neutrino oscillations. This breakthrough won a share of the 2015 Nobel Prize in Physics and changed our understanding of particle physics.
Super-Kamiokande continues to receive upgrades, and its successor, Hyper-Kamiokande, is under development. The future detector will be larger and more advanced, allowing scientists to make even more precise measurements of neutrinos and cosmic events.